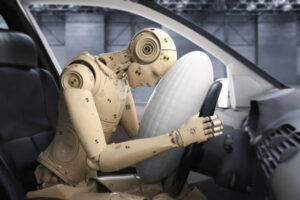
Research
Probability of Frontal Airbag Deployment in Vehicle Collisions
Accident reconstruction experts often determine the impact severity of a vehicular collision in their forensic investigations. One common collision severity index is Delta-V (ΔV). The